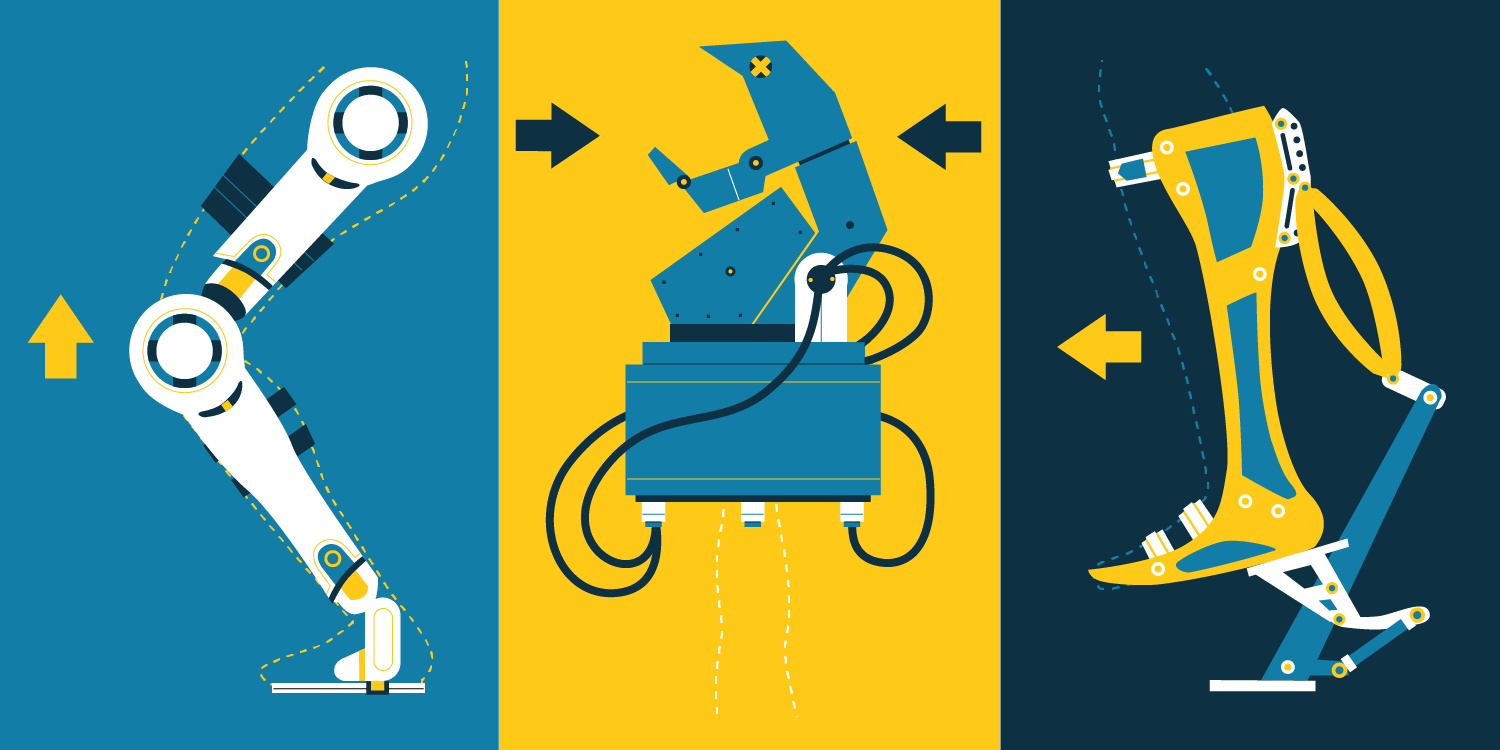
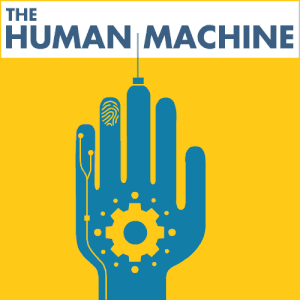
Read the next episode: “The Human Machine of 2037“
Read the previous episode: “Who Gets To Be Perfect?“

Keahi Seymour needed to get to the airport, but Manhattan traffic was gridlocked. So he ran the two-and-change miles across the island–in 12 minutes. “A taxi driver was like, “‘You beat me across the whole of Manhattan!'” Seymour isn’t a sprinter or a distance runner, but his five-minute miles were made possible with help from the Bionic Boots he’s invented, which allow him to run up to 25 miles an hour. Looking like a seven-foot-tall superhero when he wears them, he towers over the average person. “Nice robot legs!” shouted a child he passed on the street.
It’s no surprise to him that kids are intrigued by the boots–the idea for them came to him when he was a child. “I was watching a program on kangaroos, and they started to explain how a kangaroo stores energy in the Achilles tendon,” he says. At 12, he began sketching a design, looking to bipedal animals like ostriches for inspiration. Now he’s got a prototype he wears on trail runs–or just around town. “It’s an exhilarating experience,” he says. “It makes you feel like a cross between a superhero and an animal.”
Beyond exhilaration, Seymour is convinced these boots could be the next big thing. They’re a practical form of transportation, quick and compact–there’s nothing to lock up or park. You just walk–very quickly–to wherever you need to go.
If you think Seymour’s being ridiculous, consider the first cars. “People couldn’t go faster than five, ten miles an hour then,” says Seymour. “People were like, “‘Horses are more reliable,’ and then all of a sudden, it caught on. I believe we’re on [a similar] cusp of human augmentation–an exponential growth.”
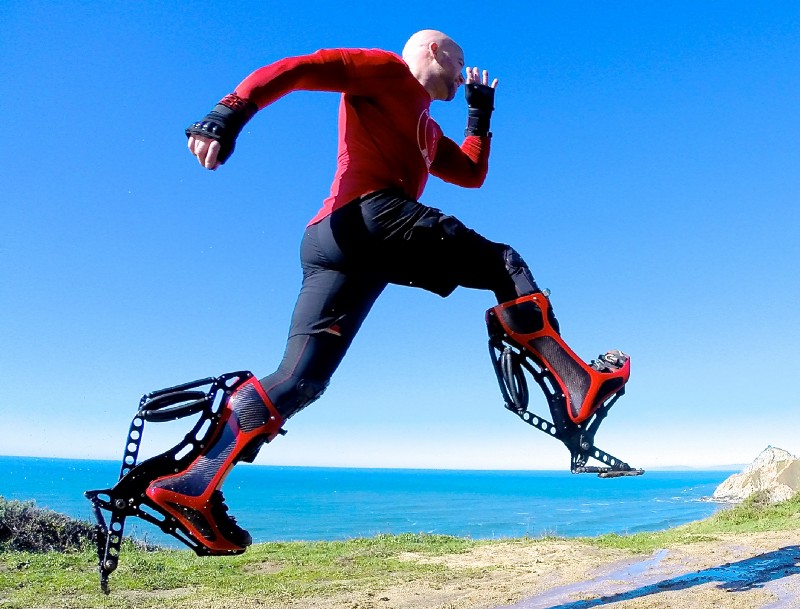
Using technology to make ourselves stronger, faster, or more efficient is nothing new. For the last 3,000 years, people have been using their eras’ cutting-edge materials–bronze, iron, wood–to replace digits or limbs congenitally malformed, lost in battle or accidents, or, like Seymour, to mimic the abilities of animals.
As our technology improves, so does our ability to propel our physical abilities to a new level. But which of us will get to be faster, or stronger?

The fastest sprinter in the world in 2068 will have an artificial limb. At least, that’s what Japanese bioengineer Hiroaki Hobara thinks. In a letter he wrote to the editor of Prosthetics and Orthotics International in 2015, he and his coauthors predicted the winning race times for future Olympics and Paralympics races. Speed times set over the last few decades indicate that sprinters using prosthetics are quickly closing in on records set by sprinters using their biological legs. Assuming this trend continues, the fastest sprinters in the world will soon be athletes with lower extremity augmentations.
We’re not there yet, of course, but we’re definitely headed there–with research into bodily mechanics paving the way. All of your movements–walking, running, lifting–require the coordination of muscle groups and tendons across your hips, legs, and feet, each making minute adjustments to account for the force of the ground, the weight of the object being lifted, and the terrain beneath the body. To figure out how to build a prosthetic that works as good as, or even better than, a biological leg, we must first figure out all of the ways that a leg works in the first place.
That’s what prosthetics company Ottobock has been doing. It focuses on prostheses for Paralympics runners and athletes competing in the Invictus Games. It also has a range of products tailored for different activities and activity levels: for professional sprinters, for people who want a prosthetic they can run errands in, for amputee soldiers getting back to work, or for elderly people who are less active. Some are even designed to be aesthetically impressive. “When I started, my patients wouldn’t necessarily want to wear shorts [and reveal the prosthetic],” Ottobock’s Chief Future Development Officer, Scott Schneider, tells me. “But now we’re putting some exotic cool looks to prosthetics–they want to talk about them, and want to share.”
Their clientele is fairly representative of the human augmentative technologies market in general, which is geared towards one of three types of people: elite athletes; soldiers and others in the military; and people with limited mobility, but personal wealth and access to medical care.
There’s a major reason why research, whether by private companies or public institutions, tends to focus on professional sports and military applications–to be blunt, that’s where a lot of the money comes from.
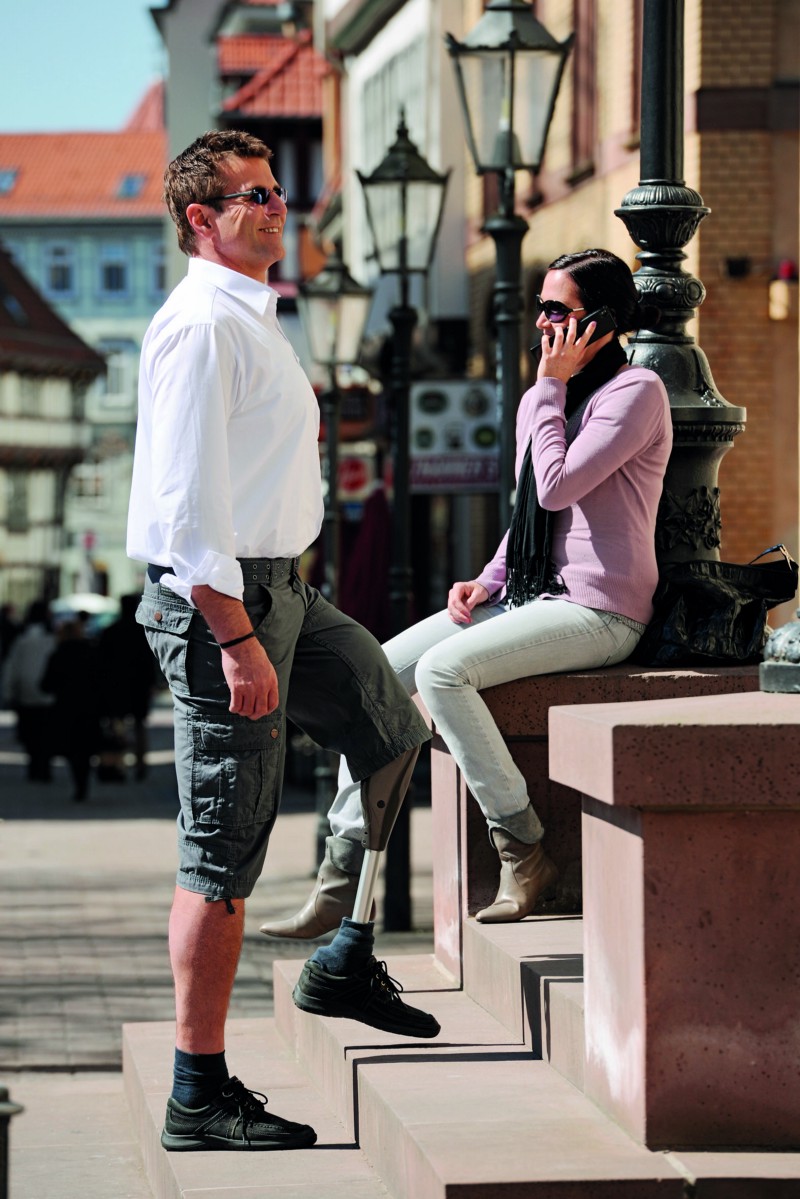
Right now, large swaths of the world’s population have only limited access to prosthetics. In the U.S., even for those with good medical insurance, advanced prosthetics are difficult to acquire–Ottobock’s C-Leg reportedly costs between $40,000 and $60,000, and it’s typical for private insurance companies to have a cap between $2,500 and $5,000 cap on prosthetic coverage. As Dylan Goldberg recounts in this piece at AlterNet, it can be extremely expensive to need something like the C-Leg–he found that merely replacing its hydraulic fluid would cost him $10,000. In countries with subsidized or universal healthcare, the need to keep overall healthcare costs lower often precludes the newest prosthetic models.
In lower-income countries, the issue is even more acute. Prosthetics there are typically made from decades-old designs and materials, or are distributed secondhand through recycling schemes, particularly when per-capita GDP might only be a fraction of the cost of even generic devices. Innovation in poorer countries tends to be focused on affordability and adaptability more than improving efficiency, power, or strength–like a project in Laos that modifies existing prosthetics using 3D printing, which Lindsey Kennedy reported on for How We Get To Next in 2015.
It’s possible that the research buoying the development of military or athletic prosthetics will eventually trickle down to the average person, like innovations for Formula 1 cars appearing, years later, in normal street vehicles. But as long as relatively wealthy sectors like the military are willing and able to pay the highest prices, many of the best new prosthetic designs will remain expensive, and unavailable to much of the public.
Independent inventors could bridge that gap, but they also face hurdles. Keahi Seymour says he’s received thousands of emails from people all around the world asking how to buy his Bionic Boots, but with so much of the human augmentation industry funded by the military or medical companies, it’s difficult for individual creators to bring new products to market without an extraordinary personal investment. Seymour, who moonlights as a bartender, has been seeking funders and collaborators for years, but has yet to find the right supporter.
Hiroaki Hobara might be right–but if the fastest sprinter in 2068 is someone with an artificial limb, the average Joe may still be stuck with technology from the 2010s.

As prosthetics improve, they’ll raise other questions about how they’re employed–especially in sports. Before South African sprinter Oscar Pistorius was convicted of murder, he was the center of a different controversy: were his prosthetic legs giving him an unfair advantage over other athletes? In 2008, the International Association of Athletics Federations barred Pistorius from competing in international competitions for able-bodied athletes, including that summer’s Beijing Olympics, citing research that Pistorius’s Cheetah Flex-Foot prostheses qualified as “technical aids.” Pistorius prepared an appeal to the decision, calling on a team of scientists to investigate the biomechanics of running with the prosthetics he used.
Alena Grabowski, now a professor at the University of Colorado, was on that team. She and her colleagues spent months studying unilateral amputees–people with one biological leg and one prosthetic–and simulated an Olympic sprint environment, in which athletes started crouched at the blocks and then took off for 200- to 400-meter sprints. They found that while the blade-like prostheses do generate some upward bouncing momentum, it was unlikely that prostheses like Pistorius’s would confer a measurable overall advantage.
Generating power is a major issue for athletes with prosthetics, and sprinters need power to build speed. “A bilateral amputee would have a 25 percent slower start than a non-amputee,” Grabowski says. Think about what it feels like to run: as your feet strike the ground, you generate downward force, creating momentum. But unlike biological legs, prosthetics don’t actively produce a force themselves. “You have to put energy into prosthetics to get energy out of them; they can’t generate power.” That’s a major disadvantage for sprinters–but the upside, says Grabowski, is that Paralympic athletes often end up adopting more efficient take-off techniques.
Blade prosthetics may not be an overall asset to sprinters, but the upward momentum they generate may enhance performance in other sports. Grabowski and colleagues have been studying their effects in long jumping, inviting professional athletes to visit their collaborators’ lab in Germany. Preliminary results suggest that while long jumpers using blade prostheses have slower approach sprints, their take-off technique conserves horizontal velocity more effectively, which could confer a slight overall advantage.
Still, though, Grabowski says it’s tricky to say whether prosthetics offer a net advantage or disadvantage in athletes’ technique or speed, since it’s impossible to systematically test pre- and post-amputation stats. And since there’s no such thing (yet!) as the opposite of an amputation, any study of an individual athlete would be biased by age–athletes are always younger before an amputation.
In the future, augmentation may take other, less detectable forms than the distinctive Cheetah-like blades. Harvard’s Wyss Institute for Biologically Inspired Engineering has been working on a new approach: a soft exoskeleton, made from textiles rather than rigid materials like plastic and metal, so that it could theoretically be worn under clothes.
Designed for use by people with conditions like multiple sclerosis or Parkinson’s, who experience difficulty walking, the soft exosuit gives a user about 30 percent more muscle capacity, says Ignacio Galiana, who manages Wyss’s Wearable Robots program. The team has taken research on bodily biomechanics and designed a network of sensors and cables (similar to those connecting a bike’s handles its brakes) that work together to mimic muscle and tendon activity.
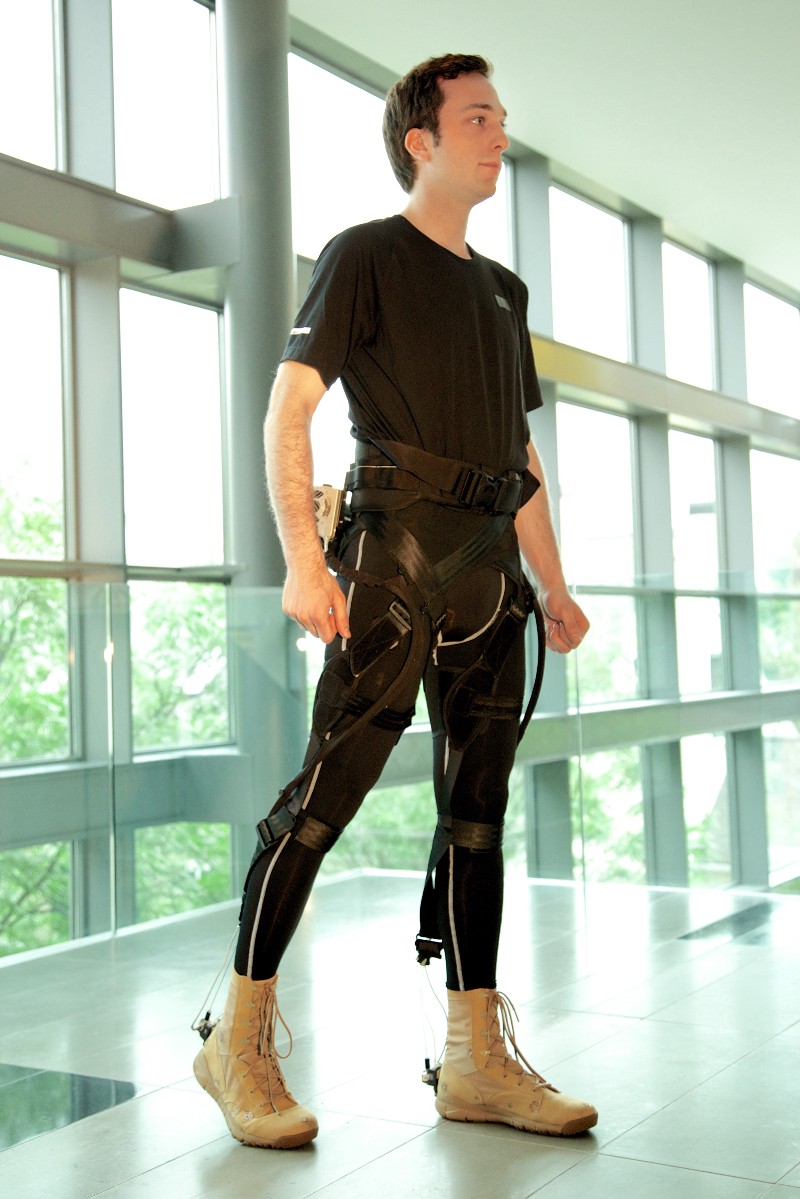
“The cables support an amount of force so that the underlying structures don’t have to do as much work,” Galiana explains. “And the sensors use controlled algorithms to detect what the human is doing, then automatically adapt to provide assistance at the right time.” As with other assistive products–hearing aids, support braces, incontinence products–wearers who would benefit from a strength-enhancing exosuit may also wish to avoid the attention that comes with a visible device.
Galiana sees wide potential for Wyss’s soft exosuits. The group is collaborating with ReWalk Robotics to bring it to market for clinical use by patients with movement disorders. But Galiana imagines that in the future, people without movement disorders may also use it, for things like boosting athleticism. “We’re going to see more wearables in the athletics field, or among the consumer and especially elderly population,” says Galiana. “You can imagine someone who’s elderly and wants to go hiking with their grandson–maybe they’ll be able to use some extra strength.”
But given that many of ReWalk’s other exosuit clinical trials have lasted from three to four years, it will likely be a while until the product is a staple for patients, let alone a commercial product available to a casual buyer.
Further down the line, Galiana says, the technology in this soft exosuit could be integrated directly into smart clothes. “Smart clothing may tell you when you’re lifting correctly or incorrectly so you don’t get injured,” he says. Or it could use your specific body mechanics, giving you personalized advice about how to improve the efficiency of your movements.
But if such sensors become embedded in normal clothing, that opens new questions for sports governing bodies: what rules or regulations could ensure that athletes are not competing with an unfair advantage? As in the recent Olympic doping scandals, tests to control augmentative technologies will also not be straightforward–and it’s an ongoing arms race between test developers and those who wish to outsmart the testers.
Galiana compares the aid one would get from a soft exosuit or smart clothing to mechanical doping in cycling, where tiny, battery-powered motors are hidden in bikes. He hopes athletes would choose to use the boost in a legal way: “They may be used to enhance training, but not to break the rules.”

So far, research into how different limbs work has been mostly used for replacing those parts. But this is changing, as technology is now advanced enough for researchers go beyond replacement, and think about full-body augmentations.
The first attempt at a full body exoskeletion was General Electric’s “Human Augmentation Research and Development Investigation,” or HardiMan, in the 1960s. It was a hulking metal creature, essentially the same idea as Sigourney Weaver’s Power Loader in Aliens. HardiMan was scrapped after engineers discovered that they had dangerously little control over the arms and risked crushing the biological arms of its operator.
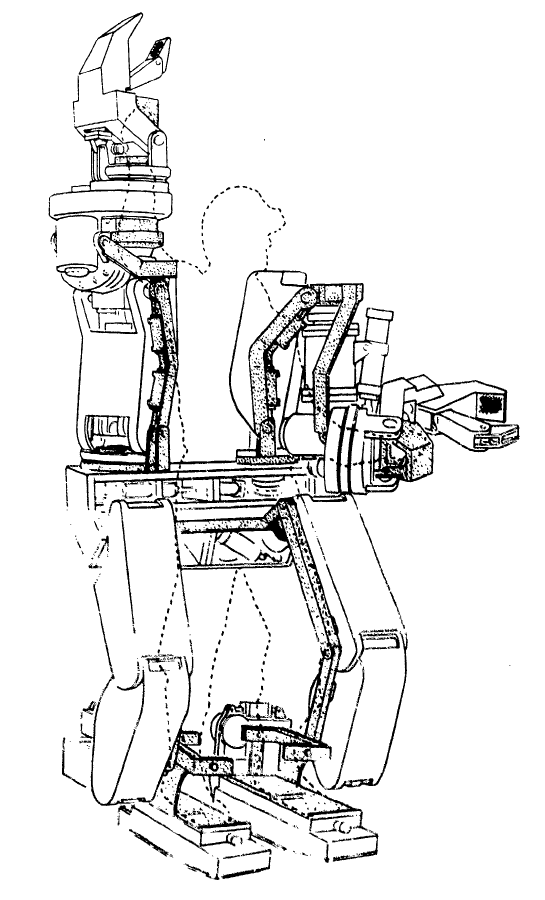
HardiMan’s successors are significantly more promising. Some, like Sarcos and Raytheon’s XOS combat suit, are designed to allow their wearer to effortlessly lift hundreds of pounds. Panasonic’s AWN-03 is designed to reduce up to 15kg of stress on factory workers’ backs when carrying heavy objects–and looks like a colorful Ghostbusters get-up. Berkeley’s Lower Extremity Exoskeleton (BLEEX) helps emergency personnel carry heavy loads, and looks like high-tech leg braces, while Cyberdyne’s Hybrid Assisted Limb (HAL–the company’s founder claims the name is a coincidence) supports the lower back and outer leg, and its white plastic makes it look like a pared-down Stormtrooper suit.
Currently there are major barriers to the mainstream introduction of exoskeletons. For starters, they have the same scalability and affordability problems as cutting-edge prosthetics–most of those research groups are based in the U.S., Europe, or Japan, and are developing applications for the military or private sector. But there are technical issues to resolve, too. While an exoskeleton might help soldiers carry more weight, or decrease the overall effort of a wearer in a factory, it will be difficult to use them to be fast.
Engineer Jason Kerestes points out that there’s a tradeoff between the amount of power an exoskeleton can provide and the amount the device itself weighs. If your aim is just to lift 50kg easily, it’s no problem for an exoskeleton to weigh that much as well–but if you’re looking to run quickly, you want something strong, while also much lighter.
Kerestes is currently at Boeing, but he spent years at Arizona State University’s Human Machine Integration Lab, designing and testing exoskeletons that pulled and pushed against runners’ leg muscles to give them a speed boost. The goal was to get soldiers running a four-minute mile. While the researchers aren’t quite at four minutes yet, preliminary tests measuring runners’ VO2-max (their maximum rate of oxygen consumption) found that the exoskeletons improved performance by 10 percent. Still, every kilogram an exoskeleton weighs has the potential to slow down its wearer. At a certain point, more power means sacrificing speed.
As part of his research, Kerestes used a golf cart test rig that pulled runners forward so researchers could observe the forces and energy needed for assisted power. “And that’s how the jetpack was born,” Kerestes says. A pair of battery-powered high-speed fans are worn around an athlete’s waist, and it uses a specific amount of power and angle of thrust–about ten percent of the wearer’s body weight, applied 25 degrees above horizontal–to push runners along. It can improve runners’ times by several seconds over short distances, and by nearly half a minute over a mile. It’s ready to go within seconds of being turned on, and the hardest part is supposedly making sure you move your legs fast enough so you don’t trip. Importantly, it provides a much better weight-to-speed ratio than other athletic exoskeleton designs.
“The biggest limiting factor is battery technology,” says Kerestes. While bigger batteries provide more power, too much extra weight reduces potential top speed. The best batteries available generate enough power for about six minutes before they need recharging; larger ones can last up to 15 minutes, but the extra weight isn’t worth the extra power. “The technology to make someone faster is here, but we’re waiting on the battery world to catch up.”
While Kerestes waits, he’s finding ways to incorporate the jetpack into activities and scenarios that could benefit from a short but powerful flight time. Extreme sports are a first obvious application. Kerestes says he’s tested the device with cyclists and has found that the boost can get a cyclist up to 50 miles an hour without pedaling. Skateboarders and base jumpers might also be interested in augmenting their sport with a quick burst of energy.
There are applications to very specific real-world situations, too. Kerestes says police officers have tried it, since the majority of foot pursuits are just a few minutes long. And the jetpack could be used for injury prevention or rehab–Kerestes says that soldiers often fracture limbs from high jumps. “The idea is that it could alleviate that impact for just a split second. Right before they hit the ground, the jetpack fires up and gets them to a safe landing speed.”

Beyond obvious, practical advantages of augmentation technology like strength or speed, they also open up new avenues for human expression. With prosthetics specifically designed for cycling, rock climbing, and archery, all kinds of sports become accessible to more people than ever before. But for Latvian-born model and musician Viktoria Modesta, prosthetics also offer the ability to express herself artistically.
At 20, after years of pain from an accident during birth, Modesta chose to undergo an operation to remove her left leg below the knee. “It’s really given me a different perspective,” she says. “What if you become more of an architect of your own physicality and identity–how much of that can you really shape, control, and decide?”
Working with designers, engineers, and scientists, Modesta’s projects turn traditional notions of disability on their head, exploring the vitality and beauty of one’s chosen body. Modesta says she’s felt a type of freedom in transcending the body, seeing it as a tool rather than a person’s defining characteristic. “I can work on designing my own limb and looking at the body as a conceptual thing or as an art piece, if I choose to,” she says. “I take strongly to the idea of redesigning my body in a way that I feel fit for the kind of story I want to tell.”
In addition to her work as an artist, Modesta is also a fellow at MIT’s Media Lab, working with researcher Hugh Herr on testing and feedback for his bionic legs, which are controlled by the wearer’s thoughts. Herr, who underwent a double amputation after a climbing accident, “has a similar vision and attitude towards wearing artificial limbs and what it means to be a person,” she says. In a 2014 interview with Newsweek, Herr went further, arguing that he’s post-human. “When you include both my biological and bionic parts, my body is evolving faster than yours.”
As the possibilities of the human body evolve, so will our perceptions of its “natural” biomechanical abilities. Modesta plays with this idea, wearing designs that specifically highlight her chosen legs: one adorned with Swarovski crystals, another lit up, attracting buzzing moths. The point is that the future of augmented human bodies could be about more than just function–it could be any number of things, provided it’s accessible and available to those who both need and want it.
“In the future, it will not be about how you appear, but how you can behave,” says Schneider. “Society’s changed; we’re moving fast, enabling people to climb, walk, crawl, and do things they’ve never done before.”

Read the next episode: “The Human Machine of 2037“
Read the previous episode: “Who Gets To Be Perfect?“
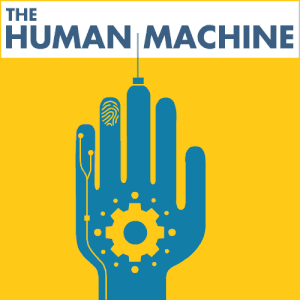
How We Get To Next was a magazine that explored the future of science, technology, and culture from 2014 to 2019. The Human Machine is an eight-part series that interrogates the increasingly blurred lines between humans and machines.